Recent advances in real-time MRI are radically changing the face of medical diagnostics, especially in dynamic processes such as speech research and cardiology. Captures are made at breathtaking rates, which allow clinicians to view, in real time, the motion occurring inside the body-something no static MRI scan could ever hope to provide. In this respect, it is similar to how MRI is used in the analysis of heart function or for tracking the movement of joints in orthopedics. Real-time MRI does, however, have unrivaled benefits regarding speech therapy and cardiac diagnostics: it increases the accuracy of interventions on a completely new level and offers direct feedback.
One of the most innovative uses of real-time MRI has to do with speech research. In a fashion quite similar to how one speaks, the moving structure of the vocal tract is captured to provide insight to the researcher and clinician about how speech is produced. This is important not only for the phonetic interpretation and the development of speech therapies but also for surgical planning, in cases where impairments of speech function or interventions at the throat are to be performed. Real-time MRI offers insights into the movements of speaking in real time, thus allowing a non-invasive way of observing the complex voicing processes that have been hardly accessible so far.
Real-time MRI has redone the definition of cardiac functional assessment in cardiology, especially in conditions of stress or exercise. It allows for the real-time visualization of heartbeats and blood flow, therefore offering critical information that may help diagnose diseases of the heart that only manifest under specific conditions. The capability for real-time capturing of cardiac motion enhances diagnostic accuracy and is of particular utility in procedures such as interventional MRI, where it guides less-invasive surgeries toward better patient outcomes and procedural safety.
Clinical Applications
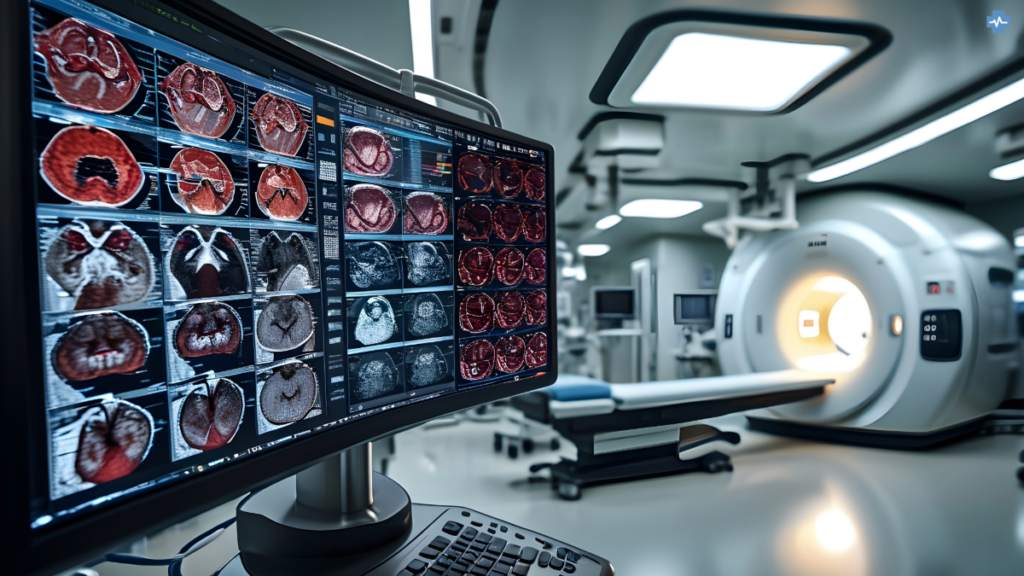
Real-time MRI would be effective only with constant improvement in the technology involved. Advanced MRI sequence designs have shortened acquisition time without affecting image quality and hence allow fast processes to be studied, such as the heartbeating or a moving tongue while uttering speech. Motion correction algorithms also help to a great extent in minimizing the artefacts produced due to the movement of the patient, which keeps scans appropriate and accurate even when situations involve dynamics.
Clinical applications of real-time MRI are manifold and growing. Real-time MRI is very important in the area of interventional radiology, guiding such complicated processes as cardiac catheterisations or needle biopsies and offering clinicians immediate feedback. This real-time guidance is crucial in navigating through complicated anatomical structures and making instantaneous adjustments during procedures. Real-time fMRI in neurology is used for finding out the exact brain areas active at any time, guiding surgeons with precision during operations to remove a tumor or carry out a surgery for epilepsy.
Real-Time MRI vs Other MRI Technology
Feature | Real-Time MRI | Conventional MRI | Functional MRI (fMRI) |
---|---|---|---|
Image Acquisition | Rapid acquisition of images in real-time | Longer acquisition times | Measures brain activity based on blood flow changes |
Applications | Cardiac imaging, interventional procedures, and dynamic physiological processes | Diagnostic imaging of various body parts | Studies brain function and neural activity |
Advantages | Allows for visualization of dynamic processes, such as heart function and blood flow | High image quality, excellent soft tissue contrast | Non-invasive assessment of brain activity |
Disadvantages | Lower image quality compared to conventional MRI, limited field of view | Longer scan times, less suitable for claustrophobic patients | Requires specialized analysis techniques, sensitive to noise |
Timeline of Key Milestones in the History of MRI
1940s – Early Theoretical Foundations
- 1946: Felix Bloch (Stanford) and Edward Purcell (Harvard) independently discovered nuclear magnetic resonance (NMR), the principle that underlies MRI. Both were awarded the Nobel Prize in Physics in 1952 for their discovery.
1950s – Early Experiments with NMR
- Throughout the 1950s, NMR was used primarily in chemical and physical analysis for studying the structure of molecules, rather than medical imaging.
1970s – Development of MRI for Medical Imaging
- 1971: Raymond Damadian, an American physician and scientist, discovered that cancerous tissue and normal tissue had different NMR signals. He proposed using NMR to detect cancer.
- 1973: Paul Lauterbur, an American chemist, published a paper on generating images using NMR, which introduced the idea of spatial encoding, a foundational concept for MRI imaging. He created the first 2D image of a living organism.
- 1975: Richard Ernst developed the use of Fourier Transform to analyse NMR signals, increasing the speed and efficiency of producing images. This was critical for practical MRI applications.
- 1977: Raymond Damadian built the first full-body MRI scanner, named “Indomitable”, and produced the first full-body scan of a human being.
1980s – Commercialisation & Clinical Use
- 1980: The first commercial MRI scanner, developed by GE Healthcare, was introduced for clinical use, marking the technology’s debut in hospitals and medical practices.
- 1984: GE Healthcare installed the first commercial MRI scanner in the UK.
- 1986: Paul Lauterbur and Peter Mansfield (a British physicist who refined MRI’s mathematical techniques to improve imaging speed) won the Nobel Prize in Medicine for their contributions to the development of MRI.
1990s – Advances in MRI Technology
- 1990: The development of Functional MRI (fMRI), pioneered by Seiji Ogawa, enabled the imaging of brain activity by detecting changes in blood flow.
- 1993: The introduction of Real-Time MRI allowed for the real-time observation of moving organs, greatly benefiting areas like cardiology and speech research.
2000s – High-Field MRI & Beyond
- 2003: Lauterbur and Mansfield were awarded the Nobel Prize in Physiology or Medicine for their work in MRI.
- 2000s: High-field MRI systems, such as 7 Tesla (T) and above, became more common in research settings, offering greater resolution for detailed brain and tissue imaging.
2010s – Advancements in High-Resolution Imaging
- 2013: The world’s first 11.7 T MRI system was developed, offering unprecedented detail in neuroimaging, significantly advancing research into conditions such as Alzheimer’s and multiple sclerosis.
- 2018: The first whole-body 7 T MRI scanner was approved by the U.S. FDA for clinical use, offering enhanced imaging for brain and musculoskeletal conditions.
2020s – AI Integration & Real-Time Applications
- 2020s: Artificial intelligence (AI) integration into MRI scans for faster image reconstruction and automated diagnostics became more widespread.
- 2024: Real-time MRI continues to advance, with applications in cardiology, neurology, and speech research, enabling dynamic, in-the-moment imaging of moving organs and functions.
Future Prospects
Real-time MRI will only continue to be a greater factor in diagnostics and procedures alike. The major drivers developing and refining MRI technology include Siemens Healthineers, GE Healthcare, and Philips, which continue to develop ways to further speed up, augment the accuracy of, and improve access to MRI diagnostics. Looking to the future of where the technology is going, real-time MRI can be combined with AI-driven analytics to enhance utility in clinical settings for more personalized and efficient care.
These advances in MRI technology offer improvements not only in diagnosis but also in the new research and therapeutic possibilities they create in medicine, such as the possibility of offering new details on dynamic processes within the body, with improved outcomes due to more appropriate treatments. However, barriers to the translation of such technologies into routine clinical practice are manifold: cost and accessibility, among others, plus specialized training to interpret the complex data arising from such modalities.